Since the start of the 20th century, physicists have discovered a veritable zoo of subatomic particles. Matter can be both wave and particle. If you take the particle route, these subatomic particles are what you could say the universe and everything in it is made of. There are many ways to further categorise them.
A common one is as fermions and bosons: fermions make up matter and bosons mediate the forces between matter. For example, electrons and protons are fermions whereas photons are bosons.
Fermions can be further classified as Dirac or Majorana fermions. Dirac fermions are fermions that may or may not have mass but are always different from their anti-particles. Majorana fermions are fermions that are also their own antiparticles (neutrinos are suspected to be Majorana fermions).

The zoo smells funny
Even if these distinctions seem too fine, they’re of considerable interest to physicists. They know something’s up in the subatomic zoo. Some animals that should obviously be there are missing, like the particle for the force of gravity. Some animals are much heavier than they should be (Higgs bosons and neutrinos). One enclosure, dark matter, remains empty even though physicists have been looking for it under every rock and leaf. Their knowledge of quite a few animals is just incomplete or at odds with what they studied in school. There’s a lot of work left if the zoo is to be a fully understood place without any surprises.
To do this physicists have one advantage: a common theory that collects all these animals under a single, unified description, called the Standard Model (SM) of particle physics. Physicists can explore ‘new physics’ in terms of whether it agrees or disagrees with the SM. Right now it’s like a big jigsaw puzzle with a few important pieces missing. If physicists find a new piece in their calculations or their particle collider experiments, they can check if it fits into the puzzle. If it doesn’t, maybe the puzzle itself needs to be changed.
In a sense, grouping fermions into fine categories is an exercise in meticulously cataloguing the exact shapes of the puzzle’s pieces. This way, if physicists find a piece whose shape is new even in a very small way, they stand to make a big update.
Something strange comes this way
A particle as it exists in the wilderness of space is slightly different from a particle that exists inside solids and liquids. “In condensed-matter physics, every material can behave like a new universe,” IIT Kanpur assistant professor Adhip Agarwala said. “Here strange particles can arise and be experimentally detectable, which are otherwise not usually seen in three dimensions.”
For example, two-dimensional materials can host particles called anyons whose properties lie somewhere between those of fermions and bosons.
Recently, physicists at Columbia University and Pennsylvania State University reported finding another strange particle called a semi-Dirac fermion.
Dirac fermions have mass and aren’t their own anti-particles. A semi-Dirac fermion has mass when it’s moving in one particular direction but not in a perpendicular direction. This unusual characteristic, which makes semi-Dirac fermions very exotic, is the result of the fermion’s interaction with the electric and magnetic forces acting on it in certain materials.
The semi-Dirac fermion reported in the experiment is technically a quasiparticle. A quasiparticle is a clump of particles or energy-packets that, in some given conditions, behaves like a single particle. Protons are quasiparticles, for example: each proton is made of three quarks and the gluons holding them together. In most settings, what separates particles from quasiparticles is a distinction without a difference. If a quasiparticle is a fermion, it’s a fermion in the same way an electron is a fermion.
Location, location, location
When trying to find puzzle pieces with new shapes, physicists need to know exactly which material to look in or they could be searching forever. This is much like in life sciences research. By studying the 1-mm-long roundworm Caenorhabditis elegans, for example, scientists have discovered many fundamental principles of biology and have won four Nobel Prizes so far. The locale of choice in the new study was a layered crystalline material called zirconium silicon sulphide (ZrSiS).

When a magnetic field is applied to a metal, the electrons inside are accelerated along a curved path. (The protons are confined to the atomic nuclei.) The energy of these electrons is called cyclotron energy.
In the metal, the cyclotron energy increases linearly with the strength of the magnetic field. This relationship can be denoted as B1, where B is the strength of the magnetic field and 1 is the exponent to which it is raised. In graphene, which is a single-layer sheet of carbon atoms linked together, the cyclotron energy increases in step with the square-root of the magnetic field strength. The relationship is thus B1/2.
In ZrSiS, the researchers found the cyclotron energy to increase as B2/3. Previous theoretical research has found that this scaling factor is a unique signature of semi-Dirac fermions.
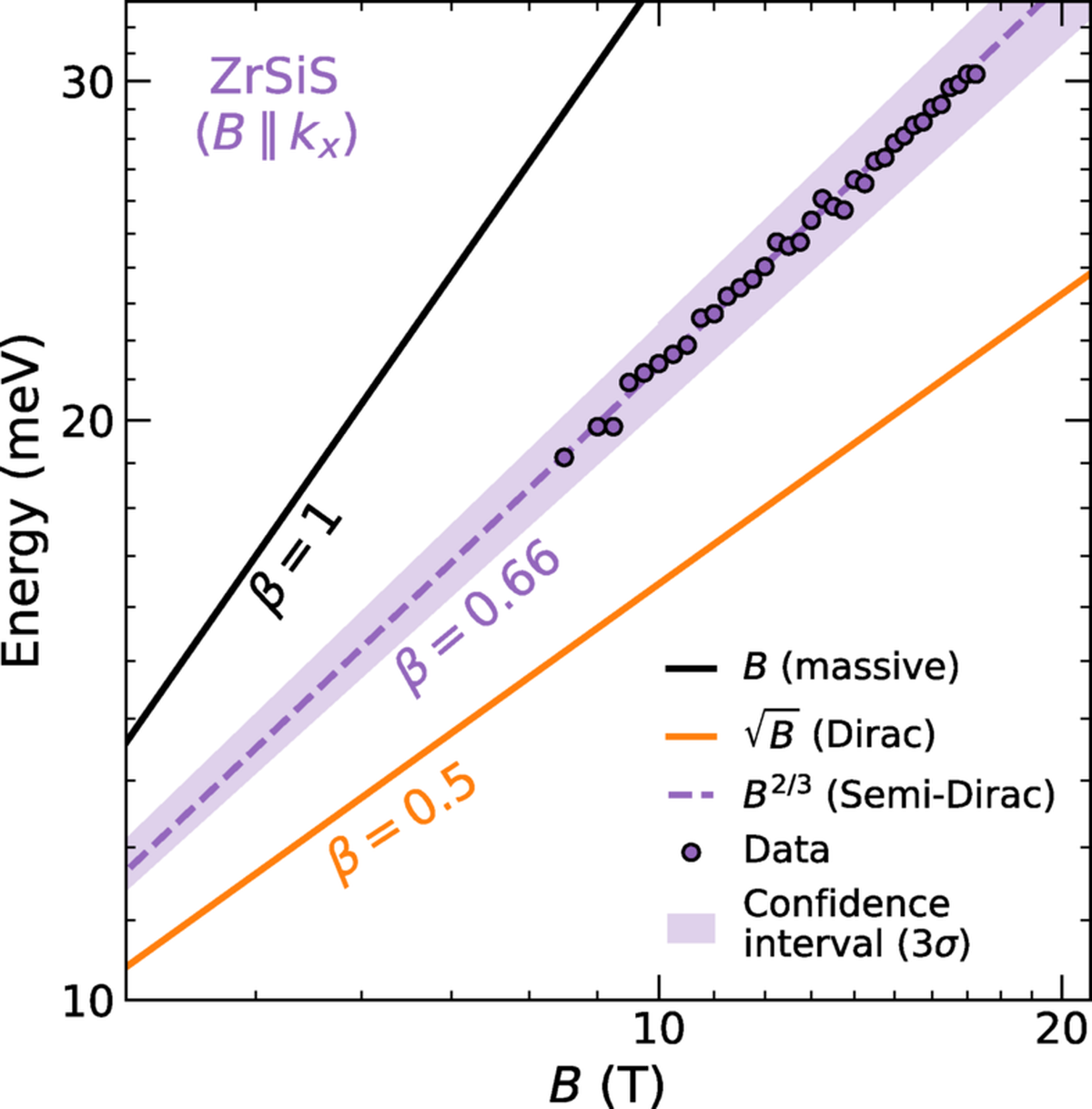
Comparison of the power law of transitions for different fermions in a log-log scale plot. Power-law fitting associated with the semi-Dirac fermions in ZrSiS shown as purple dashed line. Orange and black lines show the power-law scaling for other fermions.
| Photo Credit:
PhysRevX.14.041057
‘The same laws of nature’
The researchers didn’t land up at ZrSiS by accident; the locale is crucial, after all. Physicists in general knew for some time that there could be semi-Dirac fermions in graphene. But to reveal the quasiparticles’ presence, they had to first stretch graphene to such a degree that they often ended up tearing it apart. (Curiously, non-stretched graphene is a material that hosts a Dirac fermion, so its power law is denoted by the orange line in the graph above.) An older study also revealed some unusual electronic properties in zirconium silicon selenide (ZrSiSe), which has a similar structure, in the presence of a magnetic field.
The authors of the present study put these and other indications together and decided to look for semi-Dirac fermions in ZrSiS — and voila.
“This shows the magic of condensed matter physics, where every material, be it graphene or ZrSiS, can host exotic particles that one can discover in table-top experiments — whereas to discover subatomic particles we often need huge colliders,” Agarwala said. “It is the same laws of nature that guide them all.”

The zoo expands
Physicists regularly subject subatomic particles to extreme conditions to elucidate the laws of nature at the edge of reality. The Large Hadron Collider in CERN in Europe smashes billions of protons head on with as much energy as there was just 0.000000000000004 seconds after the Big Bang. Even in the present study, the researchers subjected ZrSiS crystals to a magnetic field of up to 17.5 tesla — about 270,000-times stronger than the earth’s magnetic field.
The researchers have said they plan to continue their studies calculations to understand more about ZrSiS and try to explain some other unusual electronic behaviour they observed in their study.
The finding is a new animal in the particle zoo. As one more enclosure awaits its occupant and zoo authorities fill out the paperwork, the question arises: how will it change the zoo?
The author thanks IISc assistant professor Nirmal Raj for feedback.
Published – January 16, 2025 05:30 am IST